Fostering Sustainable Chemical Energy Conversion Technologies through a Novel Interdisciplinary Approach which combines Combustion Science and Additive Manufacturing
High-temperature thermochemical processes combined with carbon-free chemical energy sources, such as hydrogen (H2) and ammonia (NH3), have key roles in the future energy system and the preceding transition phase. Compared to conventional fuels, H2 and NH3 have fundamentally different combustion properties, which are reflected, for example, in extraordinarily high burning rates and different flammability limits and ignition energies. In this Priority Program, SPP 2419 HyCAM, a new interdisciplinary approach is being pursued that combines combustion research and additive manufacturing competencies. The hypothesis of SPP2419 HyCAM is that only a comprehensive understanding of combustion fundamentals, as well as the integration of modern 3D manufacturing processes through simulation-based design, can enable the simultaneous improvement of flexibility, efficiency and emissions in thermochemical energy conversion processes. As a coordinated and network-building measure, this Priority Program is intended to bring together the relevant disciplines of chemistry, combustion research, materials science and manufacturing technologies. This approach facilitates the systematic, rapid, and interdisciplinary development of technology-relevant fundamentals for for advancing technology in the field of thermochemical utilization of carbon-free fuels, thereby making a significant contribution to climate protection in a timely manner.
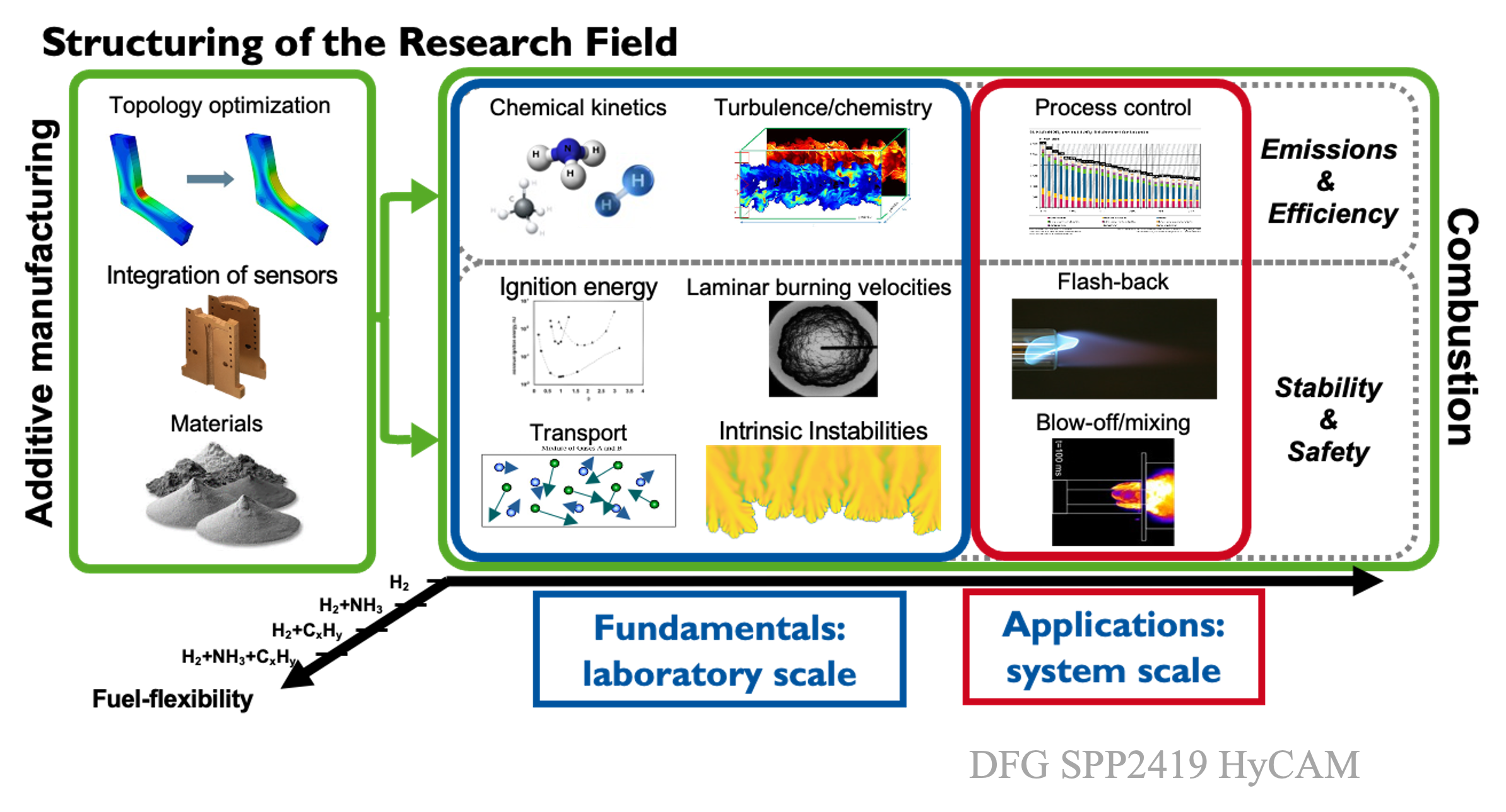
Further Information:
Thermochemical utilization of hydrogen-based fuels is essential for transforming the energy system towards carbon-neutral energy conversion, as it offers significant advantages in this context. Thermochemical energy conversion processes are widely available and reliable and can be made completely carbon-free using fuels such as hydrogen and ammonia. They are “drop-in” capable and allow gradual substitution of fossil fuels, avoiding complex, disruptive technology changes.
Therefore, high-temperature thermochemical processes combined with carbon-free chemical energy sources have key roles in the future energy system and the preceding transition phase. These technologies require fuel flexibility, which will be achieved here together with the optimization of efficiency, emissions and safety by combining combustion science with innovative manufacturing processes, e.g. additive manufacturing, and the associated degrees of freedom in materials and design. Compared to conventional fuels, hydrogen and ammonia have fundamentally different combustion properties, which are reflected, for example, in extraordinarily high burning rates and different flammability limits and ignition energies.
Due to the subordinate technological relevance of these fuels to date, many of these aspects have been insufficiently investigated and are not sufficiently well understood for further technology development. However, these fuels hold great potential for significantly improving thermal efficiencies and pollutant emissions. In this context, simulation-based design and modern manufacturing processes enable new burner geometries that can meet the high requirements when using these fuels.
In this Priority Program, SPP 2419 HyCAM, a new interdisciplinary approach is being pursued that combines the competencies of combustion research and additive manufacturing. The hypothesis of the SPP is that only a comprehensive understanding of combustion fundamentals, as well as the integration of modern 3D manufacturing processes through simulation-based design, can enable the simultaneous improvement of flexibility, efficiency and emissions in thermochemical energy conversion processes.
As a coordinated and network-building measure, the SPP is intended to bring together the relevant disciplines of chemistry, combustion research, materials science and manufacturing technologies. In this way, the technology-relevant fundamentals are to be developed systematically, quickly and interdisciplinarily so that the basis for further technology development in the field of thermochemical use of carbon-free fuels can be made possible in a timely manner, thus making an important contribution to climate protection.
Relevance of Thermochemical Energy Conversion Processes of Hydrogen-based Chemical Energy Carriers.
Hydrogen (H2) plays a special role among renewable synthetic fuels (RSFs). It can be used in industrial processes, for heating in buildings, and as fuel in transportation. Its use for time-shifted power generation is also possible. In addition to hydrogen, ammonia (NH3) can also be mentioned as a carbon-free energy carrier, the production of which is well understood and entirely possible through renewable energy sources. A significant advantage of ammonia compared to hydrogen arises from its significantly higher volumetric energy density in the liquid state, which favors long-term storage and transport over long distances.
Thermochemical Energy Conversion Processes
The energy stored in hydrogen or ammonia can be exploited through thermochemical energy conversion processes. Such processes are currently widespread in the energy sector, particularly reliable, and offer significant further optimization potentials through operation with new fuels. Relevant areas of application include back-electrification in gas turbines and, in particular, the provision of process heat using industrial gas burners. Ammonia/hydrogen mixtures are also particularly promising, as they partially compensate for the challenges in the combustion of pure substances. Potentials of thermochemical energy conversion of hydrogen also arise when mixed with natural gas, as hydrogen can be gradually added to the existing natural gas infrastructure, enabling a low-risk transition to a carbon-free energy economy. In this context, its use for space heating is also interesting. The term “hydrogen-containing fuels” refers to fuel mixtures of hydrogen, ammonia, and hydrocarbons with a high hydrogen or ammonia content.
Challenges in the use of Hydrogen-containing Fuels and the relevance of Additive Manufacturing (AM)
Molecular hydrogen is the carbon-free energy carrier closest to energy technology and mobile use. Nevertheless, there is a great need for research, mainly due to the specific molecular dynamic and flame-specific properties of molecular hydrogen, with questions regarding flame stability, flame dynamics, nitrogen oxides (NOx) formation, and safety. Potentials arise from the high burning speeds of hydrogen/air mixtures, allowing for ultra-lean combustion concepts and efficiency improvements. For ammonia, the challenges lie in the tendency to form nitrogen oxides, toxicity, which limits its use to well-monitored applications such as central industrial processes, large engines, and power generation, and combustion-specific properties such as high minimum ignition energy and low flame speeds. However, in mixtures with hydrogen, these properties can be advantageously influenced, opening up new possibilities for process control.
To optimally exploit the potential of these fuels, additive manufacturing (AM) can make an important contribution. As opposed to conventional manufacturing methods, it allows for almost complete design freedom, material combinations, and thus extensive possibilities for functional integration (e.g., sensors). This enables entirely new burner geometries to be realized, tailored to the flame dynamics of hydrogen-containing flames, which are not feasible in conventional manufacturing processes. Flame investigation and simulation results can be used for inverse design optimization. The resulting flow-optimized geometries are hardly or not at all feasible with conventional manufacturing methods. In contrast, components can be produced without the need for traditional tools in a coordinated digital and physical process chain through the use of AM and based on coupled models with the claim “form follows function”. Both entire burners or only critical components in terms of cost-effectiveness can be manufactured by AM. The challenge here lies, among other things, in processing suitable materials, which are needed due to the higher flame temperature, higher water content in the exhaust gas, and material/hydrogen interaction. New concepts for process control, such as new preheating concepts, must also be developed for realization. Furthermore, safety risks can already be addressed through component design. Further challenges lie in achievable detail resolution, resulting in surface roughness and the feedback of manufacturing-specific features (e.g., build direction, support) in the functional design process.
Structuring relevant Research Fields
It is important to understand the connection between additive manufacturing and combustion and the interrelationships in the individual disciplines.
Burner design (e.g., topology optimization), sensor integration, and materials are important for AM. For thermochemical energy conversion, the relevant processes take place on length and time scales spanning several orders of magnitude. This requires the integration of numerous disciplines on laboratory and system scales. Fuel flexibility is an aspect that plays a central role during the transition phase from today’s carbon-based energy system to a CO2-neutral future. Therefore, all thermochemical energy conversion processes require separate investigations regarding pure hydrogen, relevant mixtures of hydrogen and ammonia, and mixtures with conventional hydrocarbons.
AM can make an important contribution to all areas of combustion that need to be investigated. On the laboratory scale, specifically designed burners can be manufactured for experimental investigation, such as flame dynamics, which enable deeper insights through sensor integration or built-in gas sampling channels. Furthermore, insights from the laboratory scale can be transferred to the system scale using AM to enable the development of fuel-flexible, scalable industrial burners and gas turbines. For this purpose, fundamental questions in the areas of inverse design optimization must be addressed. Examples include digital materials with locally manipulable properties (e.g., shape memory effects), thin-walled structures (e.g., channel geometries with locally variable cross-sections), achievable surface roughness (e.g., in the area of overhangs), multiphysical topology optimization, component-integrated and/or printed sensors, as well as the processing of high-temperature-resistant materials in AM.
Goals
For a reliable and gradual transition of thermochemical energy conversion processes to hydrogen-based fuels, the necessary fundamentals and new innovative solutions through the integration of AM in burner development are to be researched interdisciplinary and cross-domain within this DFG Priority Programme. The goal is to investigate the fundamental questions of hydrogen and ammonia combustion and to establish a foundation for overcoming application-specific challenges, among others, in industrial burners and gas turbines. AM can already support this today. However, comprehensive and integrative further development of fluid mechanics and combustion fundamentals is necessary to overcome these challenges.
The SPP2419 HyCAM aims to provide insights and technologies that can be used in the short term while laying the groundwork for the long-term development of future technologies. In the short term, the knowledge gained from the SPP2419 HyCAM is intended to enable a gradual substitution of fossil fuels with hydrogen-containing energy carriers in already existing carbon-based thermochemical conversion processes, thereby paving the way for a fully ‘drop-in’ capable continuous path of energy transition. In the medium term, increased fuel flexibility and process optimization under the requirements of stability, safety, and pollutant formation are to be implemented, allowing for an expansion of the selective use of hydrogen-containing fuels. In the long term, technologies operating with carbon-free hydrogen-containing fuels are to be applied in a tailored carbon-neutral energy system of the future. For all developments, it is essential that the unique properties of these fuels, together with the opportunities opened up by the use of AM in burner design and process control, are strategically utilized to achieve targeted improvements in efficiency, emissions, and fuel and operational flexibility.
Methodic Goals
The methodological challenges in the analysis, modelling, and practical implementation of thermochemical energy conversion processes lie in the significant scale separation of physically relevant phenomena (from the molecular scale to system scale – “muti-scale“), the number of physically relevant phenomena (“multi-physics“) in combustion, and the resulting highly non-linear behavior. The conception of highly complex AM-manufactured burner concepts and coordinated operating strategies requires an integrated process using predictive simulation, AM, and experimental analysis. Methodical goals, therefore, consist of the further development of individual aspects as well as the integrative design process.
Predictive and reliable simulation models are required to optimize thermochemical energy conversion processes using inverse design simulation. However, current combustion simulation approaches often use an ad-hoc selection of models, methods, and data and do not adequately address the multiscale-multiphysics problem considered here. Model developments based on both mechanistic and data-driven approaches are therefore needed. A focus lies on quantifying uncertainties of the developed and used models.
To resolve the processes relevant to many macroscopic phenomena inside the flame structure of hydrogen-containing fuels, advanced experimental measurement methods are required, such as temporally and spatially high-resolution laser-optical techniques. Furthermore, the reduction of uncertainties in reaction kinetic parameters still represents a significant hurdle in the development of reaction mechanisms. A comprehensive reduction of model uncertainties is intended to be achieved in this Priority Program through systematic coupling of quantum mechanical calculations and experimental results. Here, the freedoms of AM are particularly advantageous in designing new fundamental experiments to reduce measurement uncertainties through sensor integration, e.g., by using printed sensors in flow reactors for measuring pressure and temperature. Furthermore, AM could provide new, cost-effective, and robust flame sensing methods, as hydrogen flames are hardly visible and ion current sensors used in many assemblies are not usable for hydrogen due to the absence of the CH radical. Additionally, high-temperature-resistant materials are integrated into the production of alloy components through rapid alloy development. The design freedom, the use of tailored alloys, and the possibilities of sensor integration through AM are intended to enable the realization of innovative concepts for the development of future highly efficient, emission-free, stable, and safe combustion systems.
Short-term Goals of the SPP (First Project Phase)
Experimental Database for Kinetic Modeling
-
Acquisition of physical insights and generation of a database from fundamental laboratory experiments and direct numerical simulations on the internal structure of reaction zones, flame stabilization, flame flashback, intrinsic instabilities, and pollutant formation; initial modeling approaches
-
Establishment of extensive, well-documented, and publicly accessible datasets for system-scale standard configurations with initial AM-manufactured burners (gas turbine, industrial burners)
-
Derivation and initial implementation of necessary development steps (design, material, process) in the field of AM (based on requirements from combustion technology) for future realization of low-emission hydrogen and ammonia combustion
-
Development of specialized, fuel-flexible, and scalable burners/turbines for experimental investigations through sensor integration and/or gas sampling channels by AM
Medium-term Goals of the SPP (Second Project Phase)
-
Predictive kinetic models for complex mixtures of hydrogen-based fuels (hydrogen and ammonia), including pollutant formation
-
Model validation for laboratory- and system-scale configurations
-
Development of hierarchical simulation methods for turbulent combustion of hydrogen-containing fuels
-
Simulative and experimental material development for AM manufacturing of combustion-relevant components (mixture, combustion, analysis)
-
Hierarchical design optimization of fuel-flexible burners manufactured by AM based on simulated and experimental investigations of flame behavior
-
Demonstration of new burner concepts on a laboratory scale through AM
Long-term Goals (Vision)
-
Automatic optimization for industrial implementation for different units with fuel flexibility up to 100% hydrogen or hydrogen/ammonia mixtures
-
Computer-aided upscaling of energy conversion plants for the energy transition (Automated) design and manufacturing of high-temperature-resistant burners using AM using multi-material processes and new concepts for temperature control of high-temperature-resistant materials (e.g., nickel-based superalloys, refractory metals)
-
Establishment of 3D-printed burner concepts on a laboratory scale, as well as automation and further development of sensor-integrated measurement technology and production of multi-material components